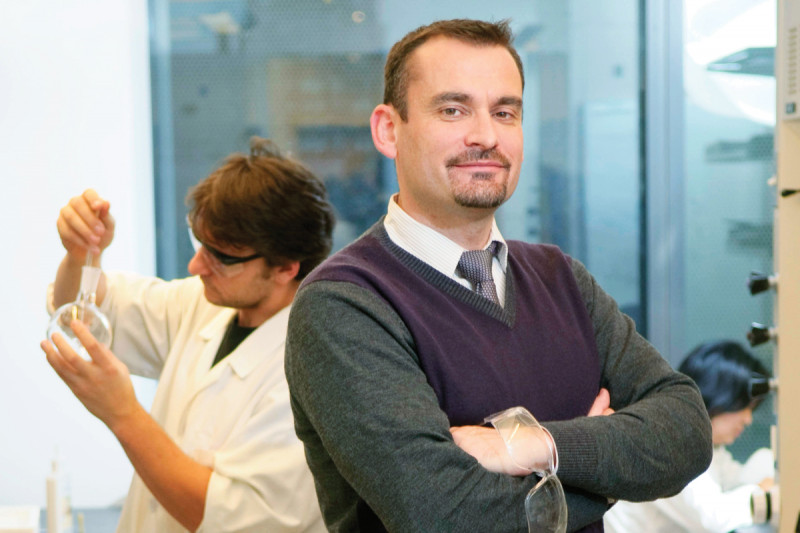
Jason Lewis
Radiochemist Jason Lewis develops radiopharmaceuticals for the imaging and therapy of cancer. In 2014, he was appointed the inaugural Director of the Center for Molecular Imaging and Nanotechnology (CMINT). We spoke with Dr. Lewis in 2008, soon after he became Chief of MSK’s Radiochemistry Service.
I come from a family of farmers and ship-builders, who live in a little village in the south of England called Horndean. A career in science wasn’t necessarily something my parents were familiar with, but they encouraged me to do whatever it was that made me happy, which, for me, was science.
In some ways I can trace my interest in science directly back to the launch of the first space shuttle in 1981. My middle school teacher at the time said that by the year 2000 anyone with a degree in science would be able to go into space. I told myself that I needed to get a science degree so I’d be ready. Needless to say, he was slightly off and I still haven’t made it into space, but that did start the ball rolling for me.
For my undergraduate degree, I went to the University of Essex, in the east of England. I chose the program because it allowed students to do a degree by thesis rather than by examination. I was much more interested in doing hands-on research than I was learning from books. My undergraduate degree was in chemistry and I narrowed the focus of my doctoral research to biochemistry, which I studied at the University of Kent at Canterbury. Specifically, I was doing research in radiochemistry, the field I work in today.
To me, what was and still is most interesting about radiochemistry is that it provides opportunities to translate agents rapidly from the research bench to the clinic. The field encompasses many different disciplines, including chemistry, biology, and physics. My PhD research involved three years of intensive radiochemistry lab work, which eventually resulted in a thesis focused on the use of radiopharmaceuticals for imaging cancer.
The Benefits of Radiochemistry
Medical cyclotrons produce the radioisotopes used in PET scanning. Radiochemistry incorporates cyclotron-produced positron-emitting radionuclides into radiochemicals and radiopharmaceuticals. In combination with PET imaging, these radiopharmaceuticals are used as diagnostic agents, designed to delineate a physiological process associated with cancer in patients. Combining radiolabeled tracers and PET imaging can provide information on functional changes well ahead of the structural changes other imaging tools can identify.
The most important feature of PET radiochemistry is that the physical amount of diagnostic or therapeutic agent that we give patients is miniscule in comparison to what is given in, for example, chemotherapy.
For example, the sugar-based radiopharmaceutical fluoro-2-deoxy-D-glucose (FDG) remains the workhorse of the PET facilities given the excellent clinical utility and the widespread availability of this drug. If you took a single FDG dose given to a patient, you would need more than one million doses to equal a single teaspoon of sugar. Because you are using such miniscule amounts, there generally is not a pharmacologically negative effect when you give radiopharmaceuticals to patients.
The other advantage involves starting with an existing nonradioactive molecule that is used as a chemotherapeutic agent, for instance, to target breast cancer. In certain situations, we can take that molecule and swap out one of its atoms for a radioactive atom. This allows us to image and track precisely where in the body and in the breast tumor that drug travels. And, moving forward, it will allow us to use noninvasive PET imaging to see how a patient will respond to therapy — before it is delivered. This, in turn, will allow us to truly tailor a treatment regimen on a patient-by-patient basis.
At a scientific conference during the final year of my PhD, I met Carolyn Anderson, who is now a professor of Radiology at Washington University School of Medicine in St. Louis. Carolyn offered me a postdoctoral position in her lab, and I jumped at the chance to work for both her and Professor Michael Welch, who, as the then-Director of Radiological Sciences at WashU, has often been referred to as the dean of radiochemistry. My plan at the time was to work with both of them for two years. But by the end of the two years, I had been offered a junior faculty position, which in turn became an assistant professor position, and I ended up staying for 11 years.
During those years, I worked on identifying small molecules to target tumor hypoxia. When certain tumors grow, oftentimes portions of the tumor are cut off from the blood supply, leaving these areas with lower oxygen levels than surrounding healthy tissues. These hypoxic tumor cells are often more resistant to chemotherapy and radiation therapy. We tested various small molecules to target these hypoxic cells. In doing so, we demonstrated that a PET-imaging-based hypoxia measurement technique using a metallic-based small molecule tracer known as Cu(II)-diacetyl-bis(N4-methylthiosemicarbazone) (Cu-ATSM) was successful in predicting a cancer patient’s response to therapy.
The hope is also that this small molecule can also be used to selectively target and kill the oxygen-deficient tumor cells. There are a number of recent papers investigating the clinical application of this molecule, and a multicenter clinical trial testing its use as a predictor of treatment response is set to begin soon.
The Lure of Translational Research
For me, the potential clinical application of my research is incredibly important. In fact, it was a major determinant in my decision, after more than a decade at Washington University, to come to Memorial Sloan Kettering, where there is such a strong emphasis placed on translational research. When I started here, it quickly became evident that Memorial is one of the leaders in bringing new radiopharmaceutical drugs into the clinic.
In addition to my joint appointments as Chief of the Radiochemistry Service and as an associate attending radiochemist in the Department of Radiology, I work closely with Dr. Steve Larson, who, as [then] Chief of the Nuclear Medicine Service, will help translate the agents developed in my lab into clinical practice. Wearing all these hats allows me to have a greater impact on clinical success. I like being able to make decisions, and I don’t mind having the buck stop with me. It helps that I have an incredibly supportive department chair in Dr. Hedvig Hricak and program chair in Dr. David Scheinberg.
The history here is remarkable. Memorial Sloan Kettering was one of the very first hospitals in the country to have its own medical cyclotron. It has been known for years for its sterling reputation in radiochemistry and translational work with PET imaging.
So far in my lab, we have a biologist, an organic chemist, an inorganic chemist, and a biochemist. It was important to me that the members of my lab had different backgrounds and expertise, which would allow them to compliment each other. To me, one of the most important aspects of being a faculty member is the training of the next generation of research scientists. It is vitally important to me that the postdoctoral fellows that come through my lab obtain a well-rounded education in all aspects of radiochemistry, molecular imaging, and translational science so that when they leave they are ready to run their own independent programs.
My lab has a number of projects ongoing. Working in collaboration with researchers from the University of Rhode Island and Yale University, one of our short-term goals is based on the discovery of the water-soluble peptide pH (Low) Insertion Peptide (pHLIP), which selectively targets cancer cells by exploiting the intrinsically low extracellular pH of tumors. We have already shown that the pH-dependent insertion of pHLIP within cell membranes allows for the targeting of tumors and the delivery of molecules to cancer cells by exploiting low extracellular pH. At the pH of normal healthy tissue, pHLIP weakly interacts with the surface of the cell membrane without insertion into it.
We have taken the pHLIP peptide and tagged it with 64Cu, and we have successfully PET-imaged tumors in vivo in mice. This agent will carry cargo across cell membranes — with these cargos having the potential to be cancer drugs or nanoparticles. It will hopefully overcome many of the delivery issues we’ve experienced with other drugs.
Another project my laboratory is focusing on is the collaboration with Steve Larson and Peter Smith-Jones, Technical Director of Targeted Radiotherapy at Memorial Sloan Kettering. Wearing my hat as Director of the Cyclotron, we are beginning to produce the PET nuclide Zirconium-89, which will be incorporated into Steve’s antibody imaging program. Members of my lab are looking into the isolation, production, and chemistry of this nuclide. Then, in tandem with Steve’s group, we will move into antibody work for hopeful translation into the clinic.