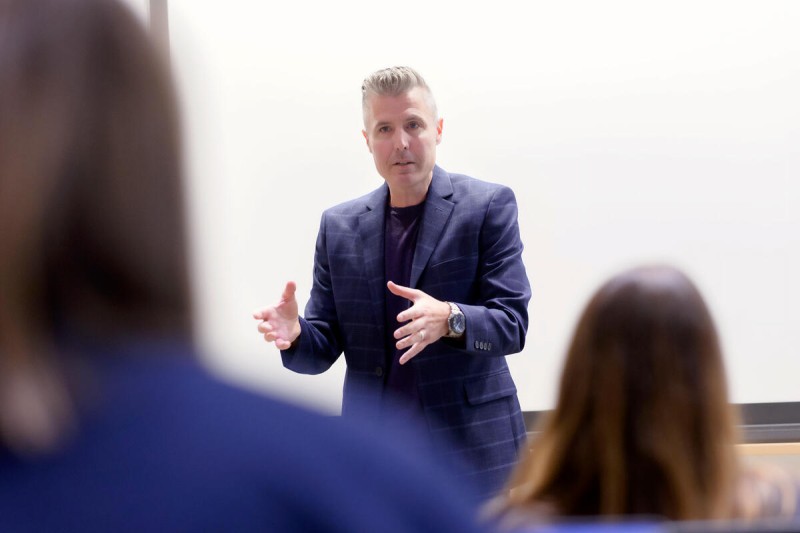
Dr. Michael Overholtzer is Dean of the Gerstner Sloan Kettering Graduate School.
On May 15, 2024, 10 newly minted PhD scientists will officially be granted their degrees from the Gerstner Sloan Kettering Graduate School of Biomedical Sciences (GSK) . The graduates will receive their diplomas as part of the 45th annual academic convocation and commencement ceremony held at Memorial Sloan Kettering Cancer Center (MSK).
The 10 trainees being honored at this year’s ceremony bring the total number of GSK alumni to 117. This year marks the school’s 13th graduating class since it admitted its first class of students in 2006.
“The accomplishments of the 2024 graduating class encompass a wide range of research subjects across the field of cancer biology,” says GSK Dean Michael Overholtzer, PhD. “Each of them has already made important contributions to the understanding of cancer, and we are excited to see what they do next.”
GSK offers the next generation of biomedical investigators a unique curriculum in cancer biology. This year GSK also launched a first-of-its-kind PhD program — the Pat and Ian Cook Doctoral Program in Cancer Engineering. The inaugural class of that program will join GSK in the fall semester of 2024.
GSK’s programs integrate MSK’s basic science and clinical arms with the goal of maximizing the potential of future scientists to solve fundamental problems in biology and to better understand, diagnose, and treat cancer.
The commencement address this year will be given by Elaine Fuchs, PhD, a professor of mammalian cell biology and development at The Rockefeller University and a Howard Hughes Medical Institute investigator. Dr. Fuchs, who studies the molecular mechanisms of stem cells, will also be honored with the Memorial Sloan Kettering Medal for Outstanding Contributions to Biomedical Science.
In 2024, GSK will award doctorates to Yu-Jung Chen, Ellen Horste, Benjamin Jackson, Yaniv Kazansky, Renhe Luo, Katelyn Mullen, Chi Nguyen, Minh Nguyen, Hongyu Shi, and Thinh Tran. Throughout the past year, these students have successfully defended their dissertations, the final requirement in earning their degrees.
The graduates describe their research projects here:
Yu-Jung Chen
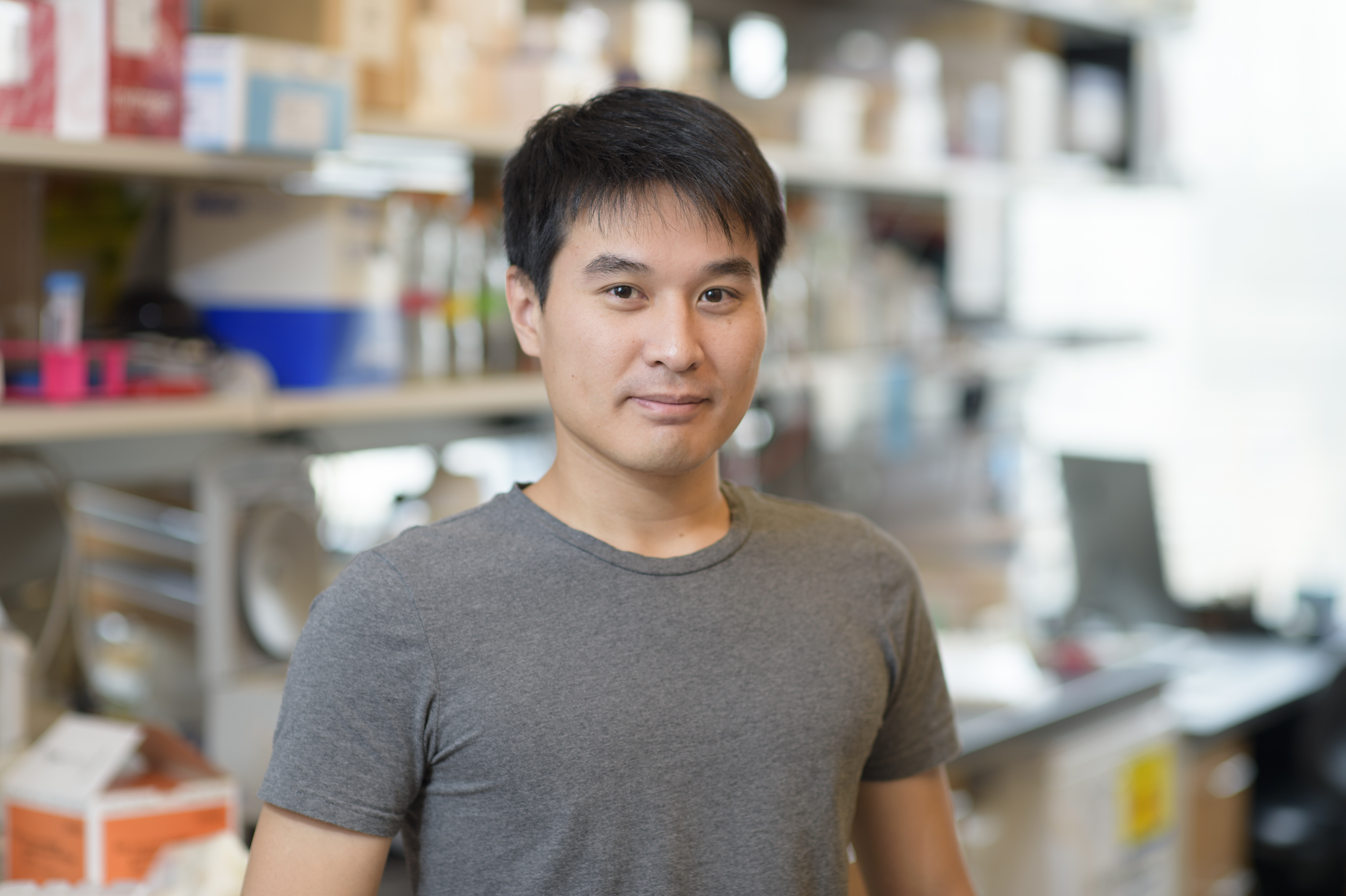
Yu-Jung Chen
I conducted my PhD research in the lab of Luis Parada, PhD, which studies the lethal brain tumor glioblastoma (GBM). My primary research objective was to explore the basic and translational aspects of GBM, with a particular emphasis on identifying potential therapeutic targets.
My major PhD research focused on the study of a novel compound, gliocidin, which targets GBM. To elucidate its mechanism of action, we performed a genome-wide CRISPR knockout screen. We discovered that gliocidin targets the de novo guanine nucleotide synthesis pathway, a crucial metabolic pathway for the rapid proliferation of tumor cells. Additionally, we elucidated that gliocidin acts as a prodrug, requiring enzymes from the NAD+ salvage pathway for metabolic activation. This dependence on NAD+ salvage enzymes provides an opportunity for patient stratification, potentially enhancing the efficacy of gliocidin treatment.
In essence, our research led to the discovery of a promising drug candidate capable of targeting GBM. Given the dearth of effective treatments for GBM in recent decades, the potential impact of gliocidin on patient treatment is substantial and warrants further clinical trial investigation.
The implication of our work extends beyond a single drug study. We revealed specific metabolic vulnerabilities in GBM and shed light on the potential to treat GBM patients by targeting tumor metabolism. However, the heterogeneous nature of GBM raises questions about the universality of these vulnerabilities across different subtypes, prompting further research into subtype-specific metabolic dependencies.
My doctoral training under Dr. Parada’s mentorship instilled in me the importance of focusing on impactful research questions within limited timeframes. Moving forward, I plan to build upon this foundation by investigating in vivo vulnerabilities of GBM using genetically engineered mouse models and patient-derived xenografts, coupled with in vivo CRISPR screens.
Attending the Gerstner Sloan Kettering Graduate School has been one of the best decisions I have made in my life. It provided me with the resources and insights needed to become a confident scientist daring to tackle difficult yet important issues in cancer research.
Ellen Horste
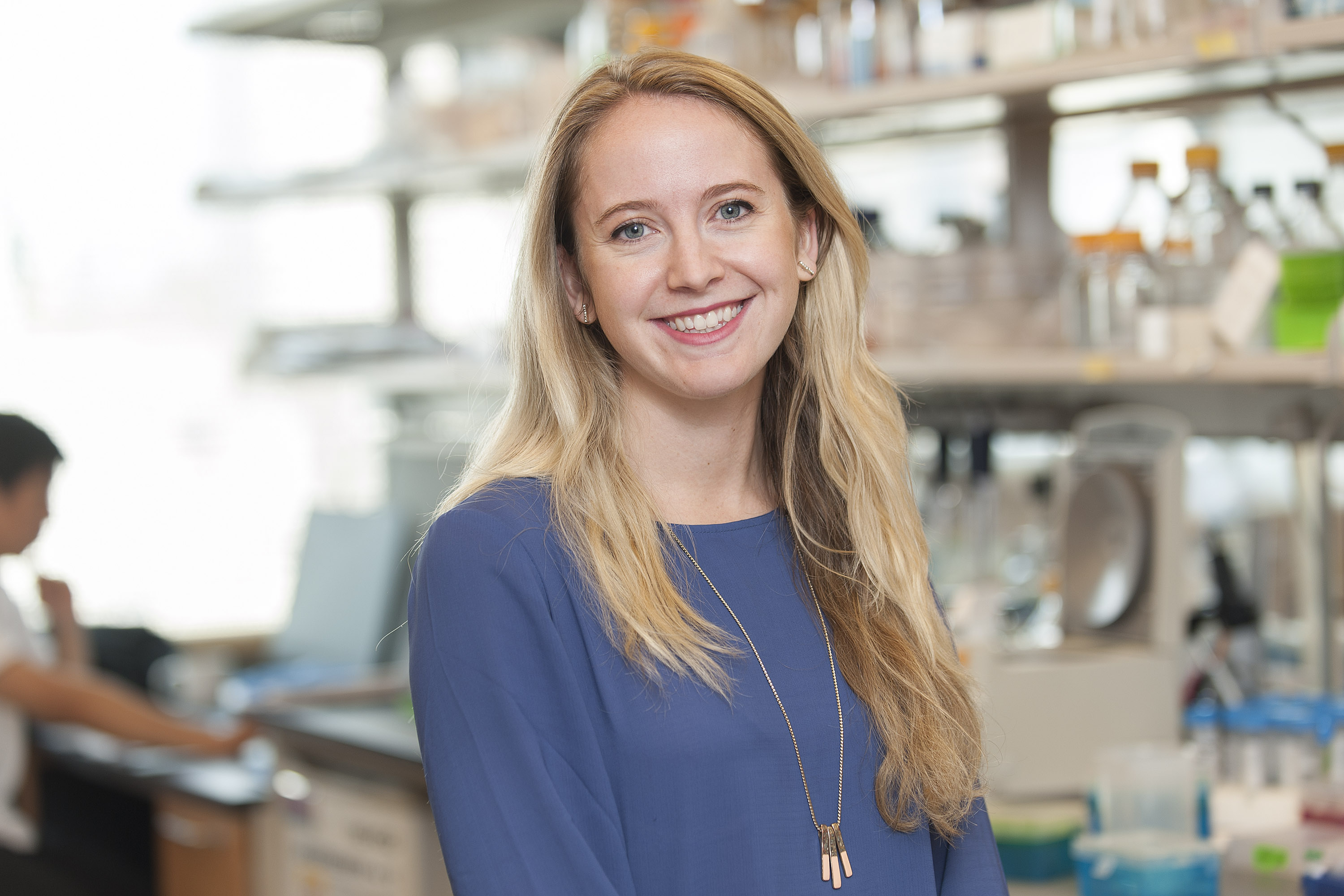
Ellen Horste
My thesis work in the lab of Christine Mayr, MD, PhD, was broadly focused on cellular organization, specifically mRNA localization in the cytoplasm. When I joined the Mayr lab, they had just discovered a novel organelle comprised of mRNAs and RNA-binding proteins intercalating with the endoplasmic reticulum (ER), but with no lipid membrane of its own.
These membraneless organelles, termed TIS granules, were observed across many cell types from multiple species, suggesting they have an important function. I was given the exciting but challenging task of characterizing these granules to investigate their function.
The foundation of this work was isolating TIS granules from cells so that we could sequence the mRNAs within. For comparison, we also sequenced mRNAs from the ER and the aqueous cytosol. Prior to this work, cytoplasmic compartmentalization beyond lipid-bound organelles was not well understood. The cytoplasm was colloquially considered by many people to be a bag of biomolecular soup, with mRNA diffusing randomly throughout. My work found this is not the case. Instead, functionally related groups of mRNAs are enriched in these three compartments of the cytoplasm, despite not being separated by lipid membranes.
Specifically, we found that mRNAs encoding proteins expressed at low levels, such as transcription factors, are translated in TIS granules. Conversely, mRNAs encoding highly expressed proteins such as cytoskeletal proteins and chromatin remodelers are translated at the ER. Through regression analysis of the mRNA features across the groups, we found that the localization pattern correlates with gene architecture features and the binding pattern of UTR-bound RNA-binding proteins.
With the recent boom of mRNA therapeutics following the success of the COVID-19 vaccines, we knew these findings could be clinically relevant, so we took the study one step further. We showed that we could control mRNA localization in the cell by tethering specific RNA-binding proteins and that simply re-localizing an mRNA to the ER increased its protein production fourfold. This localization mechanism could be leveraged in the context of mRNA therapeutics and gene therapy to modulate the protein output of a genetic payload, thus widening the therapeutic window of safety and efficacy for this emerging and transformative potential new class of drugs.
I’ve always been drawn to the innovative nature of the biotech industry, so after defending my thesis I joined Apertura Gene Therapy as a payload engineer. While the technical skills I gained in my PhD can be considered table stakes for this type of role, I’m finding that the soft skills I cultivated through participation in clubs such as the Tri-I Startup Venture Group and presenting at international conferences such as Gordon Research Conferences are just as critical to success in the fast-paced environment of a startup. I’m grateful that GSK provided and encouraged these activities.
Benjamin Jackson
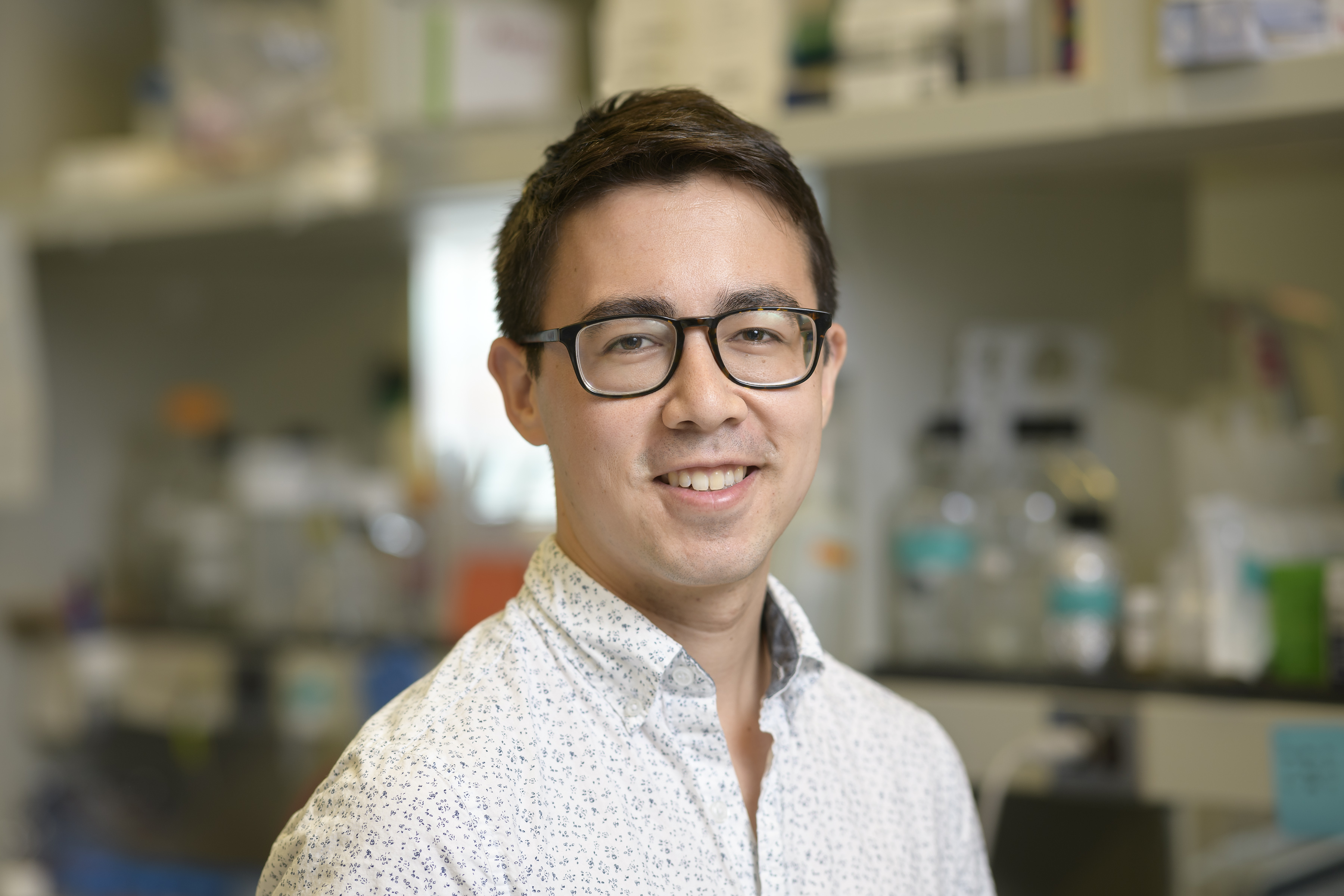
Benjamin Jackson
Cellular metabolism is the process by which enzymatic reactions within the cell break down or synthesize the macromolecules required for cellular homeostasis. Rather than being one-size-fits-all, metabolism can be flexibly engaged by different cell types in both normal development and in disease to help meet cell-type specific functions. A major goal of the field is to understand how metabolic pathways are assembled across different cells to uncover the full breadth of cellular metabolism.
During my dissertation research with Lydia Finley, PhD, in the Cell Biology Program, I focused on the metabolism of embryonic stem cells (ESCs), which are pluripotent cells derived from the pre-implantation blastocysts that are capable of forming all three germ layers of the developing embryo. During development, it is known that changes in metabolism accompany ESC differentiation. However, whether metabolism can play an instructive role to directly dictate ESC fate remains relatively unknown.
Using mass spectrometry to profile the nutrients consumed by ESCs at different stages of development, we discovered that ESCs in the naive ground state of pluripotency preferentially consume the carboxylic acid pyruvate relative to their more differentiated counterparts. Subsequent experiments identified the specific monocarboxylate transporter required by naive ESCs for pyruvate uptake and demonstrated that pyruvate is required both for regulation of cellular redox state and as a direct substrate for the tricarboxylic acid (TCA) cycle. Subsequently, removing pyruvate from ESC media or blocking pyruvate transport selectively impaired growth of naive ESCs.
Changes in nutrient uptake during ESC differentiation are accompanied by changes in nutrient utilization. In another part of my thesis work, done in collaboration with fellow GSK alumn Paige Arnold, we revealed that during the exit from naive pluripotency, ESCs switch from engagement of the canonical TCA cycle and instead utilize an alternative “noncanonical” TCA cycle to satisfy the bioenergetic demands of differentiation.
Furthermore, this noncanonical TCA cycle is active in cancer cells in culture and underlies other cell-state transitions such as muscle differentiation. These findings highlight the utility of basic metabolism research to uncover new pathways that might have therapeutic relevance to the treatment of human disease.
As an MD-PhD student, GSK has been an ideal graduate program to witness the intersection of basic science and translational biomedical research. Having completed my PhD, I am currently in the final stages of medical school, and plan to apply for residency programs later this year to begin the next step of my clinical training. Ultimately, my goal is to combine medical practice with research to probe the mechanisms by which metabolic regulation and dysregulation underlies human physiology and disease.
Yaniv Kazansky
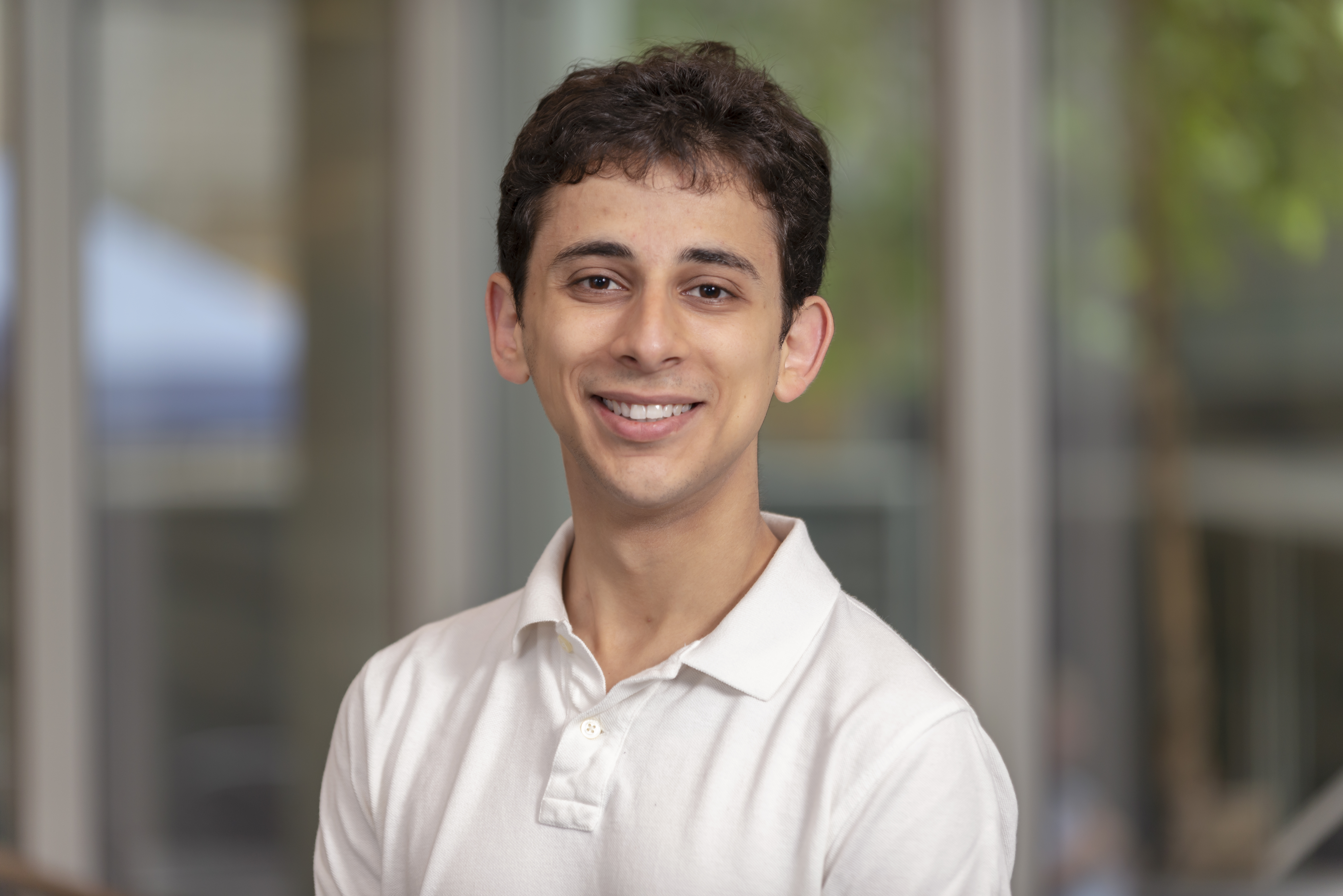
Yaniv Kazansky
I conducted my thesis research in the lab of physician-scientist Alex Kentsis, MD, PhD. The research in Dr. Kentsis’s lab is inspired by the children and young adults that he treats at MSK. My research focused on pediatric sarcomas, deadly solid tumors that mainly strike children and are often driven by just a single mutation. These mutations are powerful enough to profoundly dysregulate gene expression and transform cells.
However, I believe that they also create a therapeutic opportunity: If we can target the single fundamental defect in these cancers, we have the potential to impair the cancer just as profoundly. Still, while the causative mutations in many pediatric sarcomas are known, targeted therapeutic strategies are lacking, and the prognosis for patients remains dismal. Furthermore, our understanding of the molecular mechanisms that allow these mutations to transform cells is incomplete, hampering the development of targeted therapies.
A subset of pediatric sarcomas, including malignant rhabdoid tumors and epithelioid sarcomas, are caused by loss of the SMARCB1 gene. These tumors depend on the activity of the gene repressor EZH2. Excitingly, the EZH2 inhibitor tazemetostat was recently approved by the Food and Drug Administration as the first targeted therapy for these tumors. However, most patients do not respond to tazemetostat, and there is a critical need to better understand the principles of tumor response and resistance EZH2 inhibition.
In my work, I showed that many tumors evade tazemetostat through dysregulation of a common cell cycle control pathway. I proposed and successfully tested two combination strategies to circumvent tazemetostat resistance and identified ways to predict drug response. This offers a paradigm for rational epigenetic combination therapy that can be quickly brought to patient trials.
I also investigated Ewing sarcoma, a bone tumor caused by the EWS-FLI1 fusion, which works by turning on inappropriate genes across the genome. The precise molecular mechanism by which the fusion protein drives oncogenic gene expression is unknown. Using mass spectrometry and genetic dependency analysis, I defined the complete interactome of EWS-FLI1, discovering exciting new cofactors. Then, using a peptide interference approach, I created a novel peptide-based molecule that reduces the expression of EWS-FLI1 target genes. In future work, this approach should form the basis of new therapies to combat this devastating disease.
Overall, my work demonstrated and advanced multiple strategies for targeting pediatric sarcomas based on new knowledge about the basic biology of these tumors. As I next complete medical school and residency, I hope to apply the valuable skills I learned in the Kentsis lab as a physician-scientist, working in both the laboratory and the clinic to find new ways to treat patients with devastating diseases.
Renhe Luo
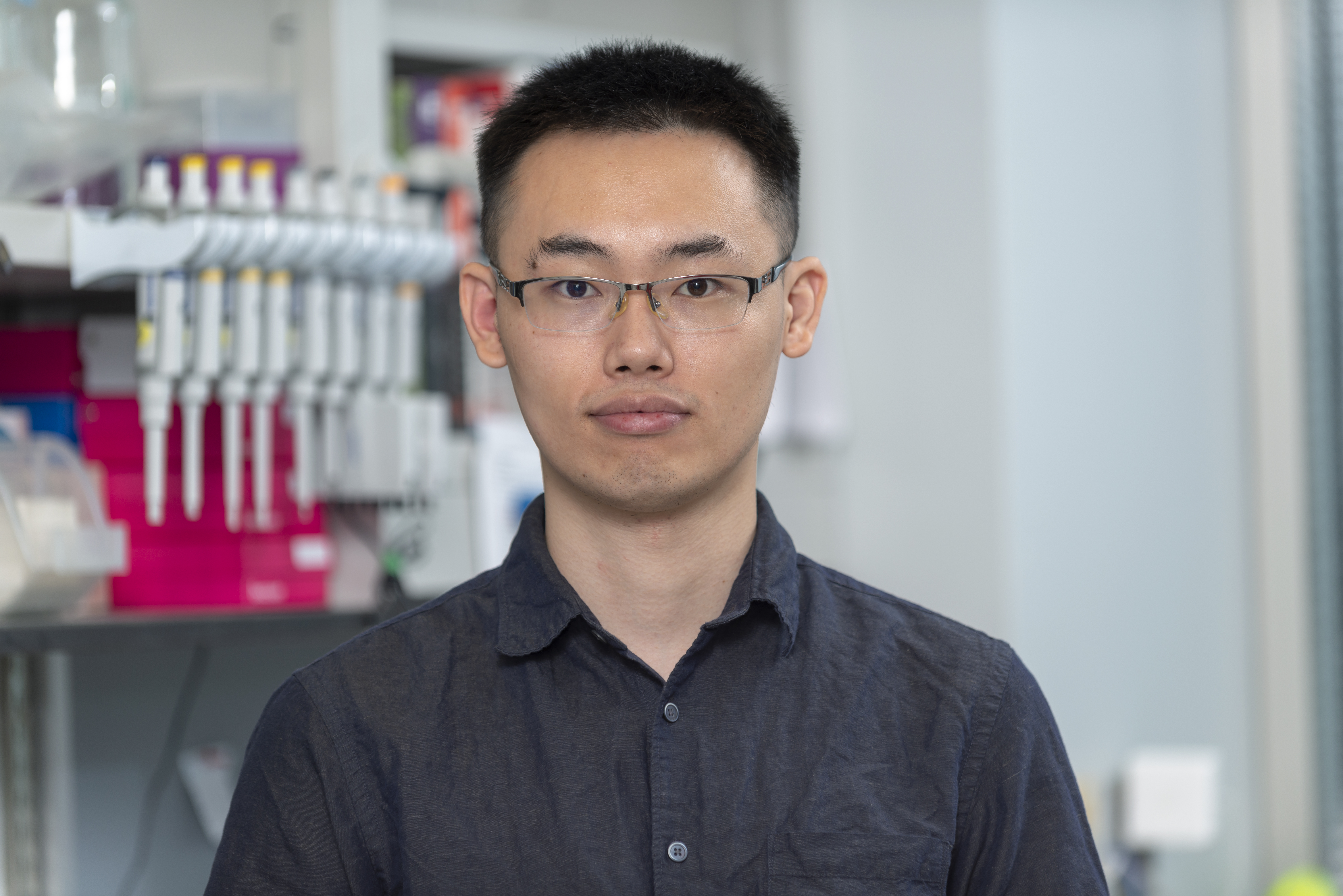
Renhe Luo
Gene regulation, a sophisticated and dynamic process, intricately orchestrates the interplay between proteins, DNA, and various molecules to finely modulate gene expression within cells. This intricate dance is critical for fundamental biological phenomena such as development, tissue homeostasis, immune responses, and adaptation to environmental changes.
Within this regulatory ballet, enhancers emerge as specific DNA sequences facilitating the binding of transcription factors and other regulatory molecules. These enhancers form essential bridges, connecting different genes within a gene regulatory network (GRN). Therefore, the mapping of enhancers in a GRN is pivotal for unraveling the mechanisms of gene regulation.
My thesis work in the lab of Danwei Huangfu, PhD, centered on the mapping entire enhancer atlas of a GRN with improved enhancer discovery efficiency using novel technologies. Comprehensive enhancer discovery is challenging because most enhancers, especially those affected in complex diseases, have weak effects on gene expression. To address this, I employed dynamic network modeling, revealing that nonlinear enhancer-gene regulation during cell state transitions can enhance the sensitivity of enhancer discovery.
Guided by these modeling insights, a pooled mid-transition CRISPR interference-based enhancer screen was conducted to map enhancers for lineage-specifying transcription factors (TFs). This approach utilized the dynamic transition from human embryonic stem cells to definitive endoderm as a model system. The screen identified a comprehensive set of enhancers of endoderm lineage-specifying TFs, including those with robust effects during cell-state transitions but moderate effects post-transition. To complete the enhancer atlas of the endoderm GRN, a genome-scale single-cell perturb-seq enhancer screen was performed, identifying over 300 regions whose perturbation significantly decreased the expression of target genes.
In summary, our gene regulatory network hierarchy concept, coupled with dynamic network-guided enhancer screens, provides generalizable strategies for sensitive and comprehensive enhancer discovery. These approaches are applicable not only in normal cellular transitions but also in pathological contexts.
The exposure to cutting-edge technologies such as gene editing, CRISPR screens, and single-cell sequencing during my PhD journey has fueled my excitement and commitment to this field. As a research scientist in the vector optimization team at Spark Therapeutics, I am applying these groundbreaking technologies to gene therapy, potentially reshaping the future of patient care through innovative gene therapy approaches.
Katelyn Marie Mullen
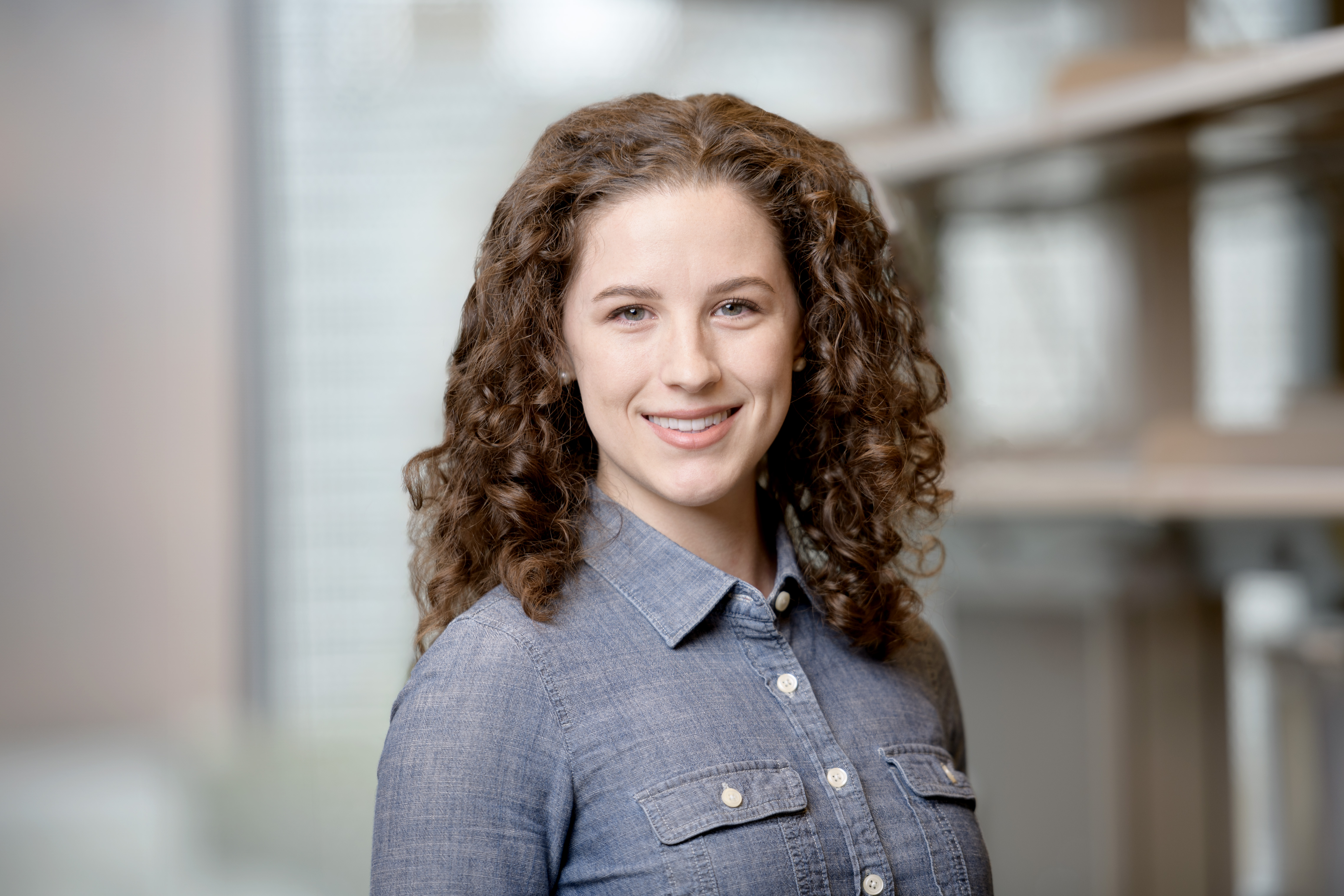
Katelyn Marie Mullen
I conducted my dissertation research with Christine Iacobuzio-Donahue, MD, PhD, a member of the Human Oncology and Pathogenesis Program and Director of the David M. Rubenstein Center for Pancreatic Cancer Research. Dr. Iacobuzio-Donahue studies the genomics and cell biology of pancreatic cancer as it relates to subclonal evolution, tumor progression, and metastasis. Her laboratory is unique in that it utilizes primary and metastatic tissues obtained through post-mortem donations from cancer patients in combination with next-generation sequencing of the harvested tissues to understand the mechanisms of metastasis.
My thesis work focused on understanding the genomic landscape and clonal composition across the full spectrum of clinical disease contexts in pancreatic cancer. Intratumoral heterogeneity, which refers to distinct tumor cell populations within the same patient, has been associated with cancer progression and is thought to be a major contributor to treatment resistance. However, the extent to which different genetic drivers that arise during carcinogenesis specifically influence subsequent evolutionary trajectories and clinical course is an active area of research.
I found that the timing of driver mutations, meaning those that happened earlier versus later in tumor evolution, did not differ significantly across clinical contexts. However, having more mutations occur earlier in tumor evolution was significantly associated with worse overall survival after adjusting for stage at diagnosis, age, and smoking history, representing a potential prognostic biomarker in this tumor type. I also found that treatment status and disease stage were associated with the clonal composition of pancreatic ductal adenocarcinoma — with treated and late-stage patients having increased odds of being polyclonal.
I chose GSK for my PhD studies because of its unique program structure and close-knit community. Selecting a lab is a significant decision, and I really appreciated how the graduate school acknowledged this by keeping rotations and coursework separate so that students can fully engage with the task at hand in order to make the best choice. I am confident that the critical reasoning skills I honed during my time at GSK will serve me well in my next role as an Informatics Specialist II at MSK.
Chi Nguyen
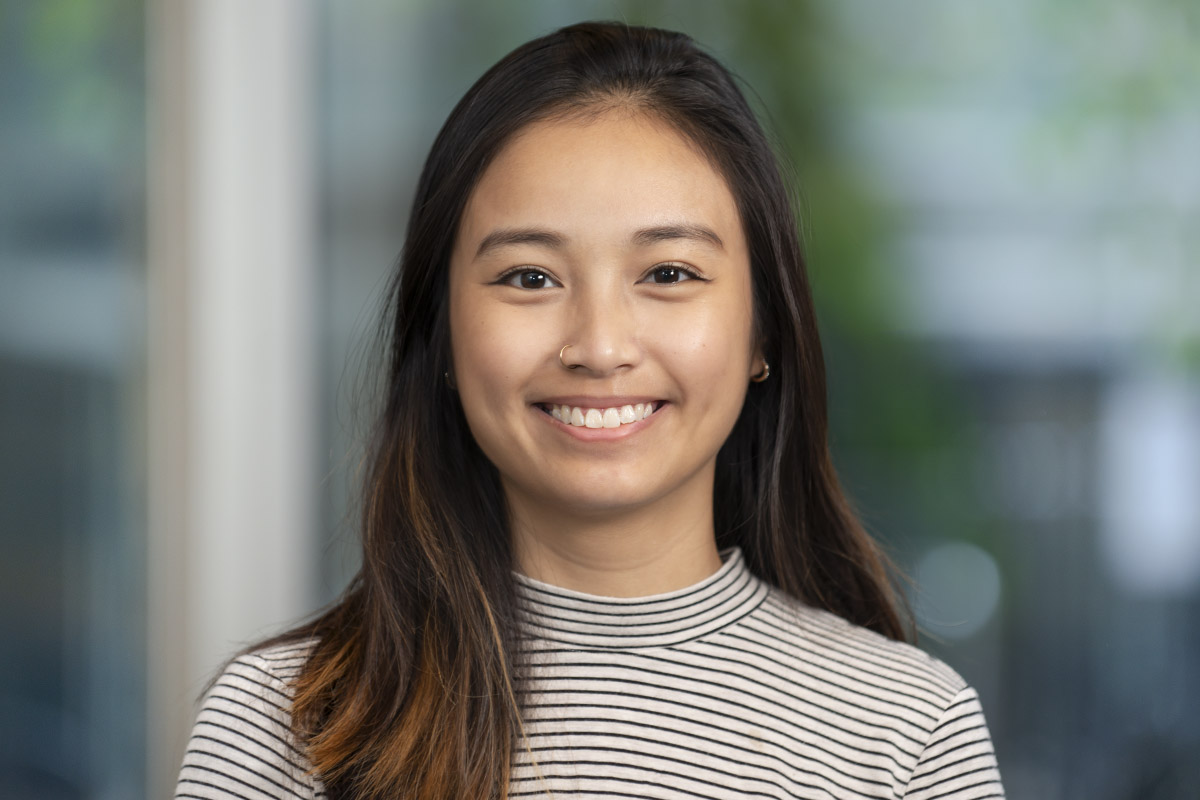
Chi Nguyen
I completed my dissertation research in the laboratory of Marcel van den Brink, MD, PhD. The van den Brink lab investigates novel strategies to improve cellular therapy, with a special emphasis on the role of the intestinal microbiome, chimeric antigen receptor (CAR) T cell therapy, and regeneration of thymic functions and T cell immunity.
The first part of my dissertation sought to understand the interactions between pharmacological exposures and the intestinal microbiota in the setting of allogeneic hematopoietic cell transplantation (allo-HCT). Allo-HCT is a potentially curative treatment for patients suffering from various hematological malignancies. However, post-transplant complications such as graft-versus-host disease (GVHD) remain significant mortality risks for allo-HCT patients.
Gut microbiome dysbiosis has been linked to poor survival and increased GVHD-related mortality risk following allo-HCT. Understanding how environmental factors such as pharmacological exposures affect the gut microbiota would pave the first step towards optimizing clinical decisions to preserve gut microbiome health in order to maximize patient outcomes following allo-HCT.
To answer this question, we developed a novel computational model, termed PARADIGM (PARameters Associated with DynamIcs of Gut Microbiota) and applied it to a large dataset of longitudinal microbiome sequencing profiles and medication exposures. We demonstrated that several non-antibiotic medications, such as laxatives, antiemetics, hormones, and opioids, are associated with changes in the gut microbiota (such as increased Enterococcus relative abundance or decreased biodiversity).
We found a significant concordance between our in silico results and published measurements of in vitro antibacterial activities for a subset of investigated medications. Importantly, we demonstrated that drug-microbiome interactions could reliably predict future microbiome trajectories and clinical outcomes such as overall mortality and GVHD-related mortality. Altogether, these data suggest that non-antibiotic pharmacological exposures could potentially shape the gut microbiota, thus bringing forward hypotheses regarding medication-microbiome interactions that could be utilized to inform clinical practices. The discoveries of this study were published in Cell.
The second aim of my dissertation attempted to delineate the immunological mechanism by which bacterial genus Enterococcus aggravates GVHD pathophysiology. Our human associative studies have demonstrated that intestinal Enterococcus domination is associated with increased GVHD-related mortality. We focused on one specific immunological pathway driving T cell activation, which is enhanced antigen presentation by non-hematopoietic cells such as the intestinal epithelial cells (IECs). Antigen presentation via major histocompatibility complex class II (MHC-II) plays a particularly important role in GVHD pathophysiology.
Using mouse models of GVHD, we observed that endogenous Enterococcus abundance was significantly correlated with increased expression of MHC-II by IECs during GVHD. Moreover, colonization of gnotobiotic mice with Enterococcus was sufficient to induce MHC-II expression by IECs at steady state, while colonization with other Gram-positive bacteria such as Blautia did not affect colonic epithelium MHC-II expression. Further work is necessary to identify specific immunogenic factors from Enterococcus that drive its interactions with the gut epithelium.
I was drawn to GSK because of its unique focus highly translational cancer research and emphasis on collaboration. I had the opportunity to interact closely with computational biologists, bench scientists, and physicians, which enhanced my learning across different disciplines and improved the quality and clinical relevance of my research. I will use the interdisciplinary skills I have gained through my time at GSK in my future professional journey.
I am currently continuing my second dissertation aim in my current lab at MSK as a research fellow. Upon publication of my research and completion of my postdoctoral training, I will transition to a life science specialist position at L.E.K. Consulting in Boston, where I will contribute to life science and healthcare innovations through strategic advice to biopharma companies.
Minh “Evelyn” Nguyen
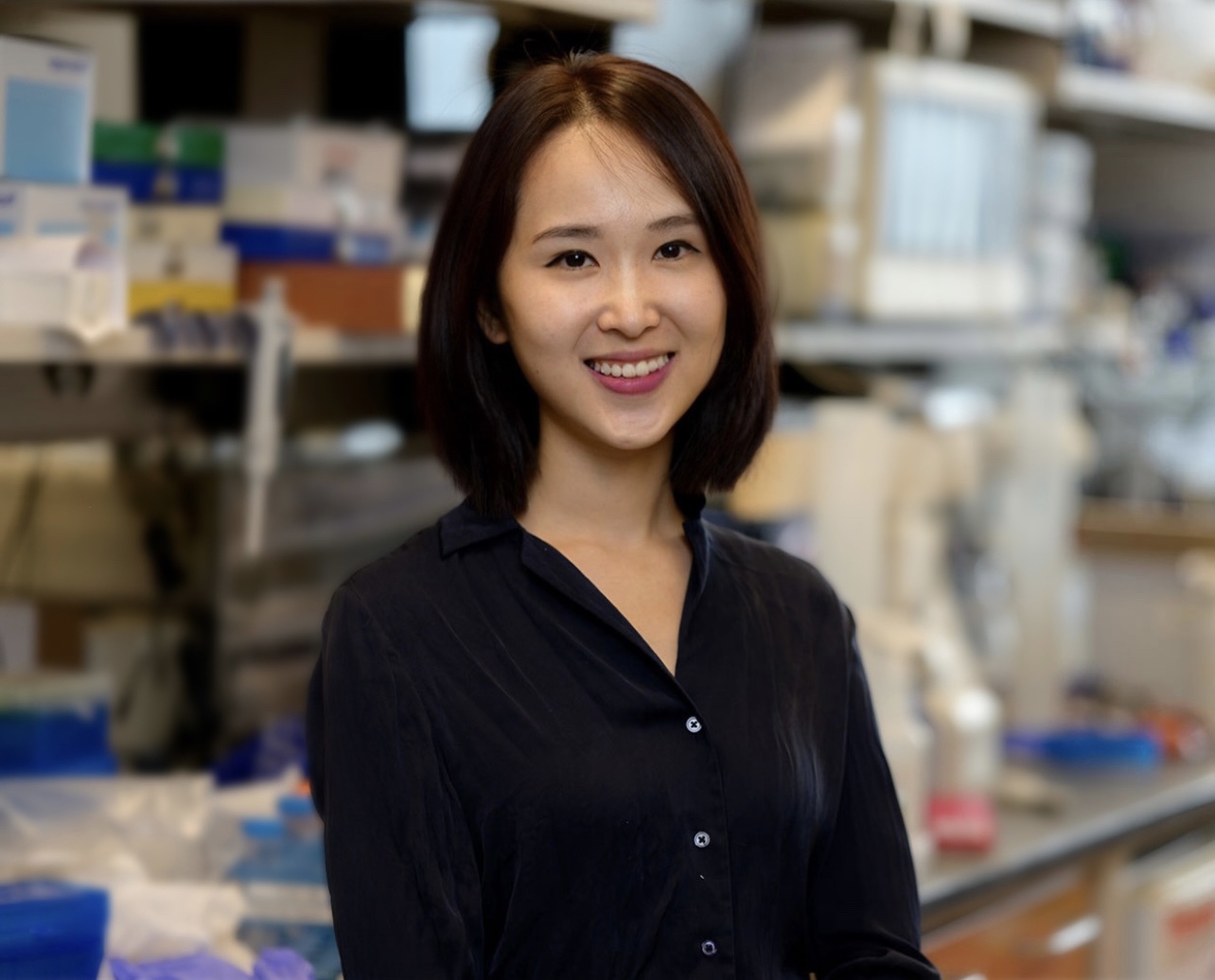
Minh “Evelyn” Nguyen
I completed my dissertation research in the laboratory of Charles Rudin, MD, PhD, Chief of the Thoracic Oncology Service and Cancer Center Deputy Director. The Rudin lab focuses on the development of novel therapeutic approaches to lung cancers using patient-derived xenografts (PDX) and other preclinical models.
Immunotherapies engage the patient’s own immune system to eliminate cancer cells. This approach has revolutionized treatments for many solid tumors but not for small-cell lung cancer (SCLC), a highly lethal lung cancer type. Central to immune surveillance is the ability of tumor cells to exhibit MHC-I molecules that display a snapshot of the tumor aberrant self to the immune system. In SCLC, MHC-I is severely downregulated, rendering the tumor “invisible” to T cells. My thesis work sought to understand the mechanism of MHC-I deficiency and develop novel combinational therapies to overcome this resistance.
My first project identified signatures associated with immunotherapy sensitivity in SCLC patient tumors and illustrated the complex functions of the tumor microenvironment in driving durable clinical benefit. MHC-I expression was the most significant tumor biomarker associated with immunotherapy response, yet the rate of MHC-I loss is significantly lower than that observed in other lung cancers. This study decodes novels mechanisms of immune escape in SCLC and furthers our understanding of how SCLC tumors might resist immunotherapy.
Next, I investigated whether MHC-I expression can be therapeutically rescued through targeting the epigenetic modifier LSD1. We showed that pharmacological inhibition and genetic perturbation of LSD1 re-expressed surface MHC-I, enabled cognate T cell lysis and sensitized resistant SCLC tumors to anti-PD-L1 blockade. Our study nominated LSD1 as a novel driver of MHC-I antigen presentation and a promising candidate for combinational immunotherapies.
In my last project, I performed a “druggable” CRISPR screen to discover regulators of MHC-I that can be readily pharmacologically targeted. This genetic screen decouples MHC-I regulation from canonical interferon activation and identified previously unexplored regulatory pathways in MHC-I antigen presentation.
I chose GSK for my PhD training because of the translational focus and the rigorous interdisciplinary curriculum that cultivates an exceptional intersection of research and patient care. I am bringing this impact-driven mindset in the next chapter of my career as a consultant at Boston Consulting Group, where I help provide strategic advice to the life sciences and biopharma industries.
Hongyu Shi
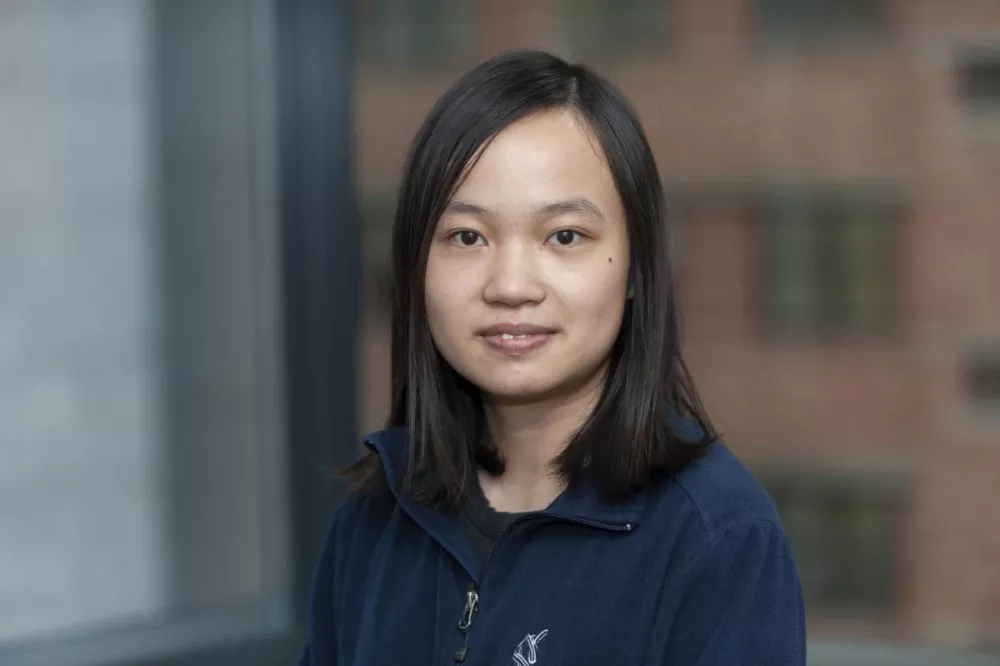
Hongyu Shi
Genomic instability is a hallmark of human cancer that leads to copy-number alterations (CNAs) in cancer cell genomes and extensive intra-tumor heterogeneity. Previous studies have shown that CNAs can affect how genes are expressed, but it is not always straightforward to understand how CNAs change gene expression in subpopulations of cancer cells within the same tumor. This is where my research, conducted under the mentorship of Sohrab Shah, PhD, and Nikolaus Schultz, PhD, comes in.
We investigated how CNAs drive gene expression differences in cancer cells. Our new method, called TreeAlign, helps us understand this by looking at both the copy number alterations and the expression level of genes within single cells. TreeAlign can integrate independently sampled single cell DNA (scDNA) sequencing and single cell RNA (scRNA) sequencing data in a biologically meaningful way. By doing this, we can pinpoint which genetic changes are responsible for specific expression dysregulation in subpopulations of cancer cells.
We’ve tested TreeAlign extensively and found that it outperforms other methods in accurately integrating scDNA and scRNA datasets and predicting how genetic changes affect gene activity. By applying TreeAlign to data from ovarian cancer patients, we’ve uncovered diversity of gene expression profiles in ovarian cancer cells and how CNAs contribute to these differences.
Overall, our research shed light on the complex relationship between CNAs and gene expression in cancer cells. By understanding these relationships better, we hope to find new ways to target the heterogeneous cancer cells more effectively in the future.
Since graduation, I have been working as a machine-learning software engineer at Benchling Inc.
Thinh N. Tran
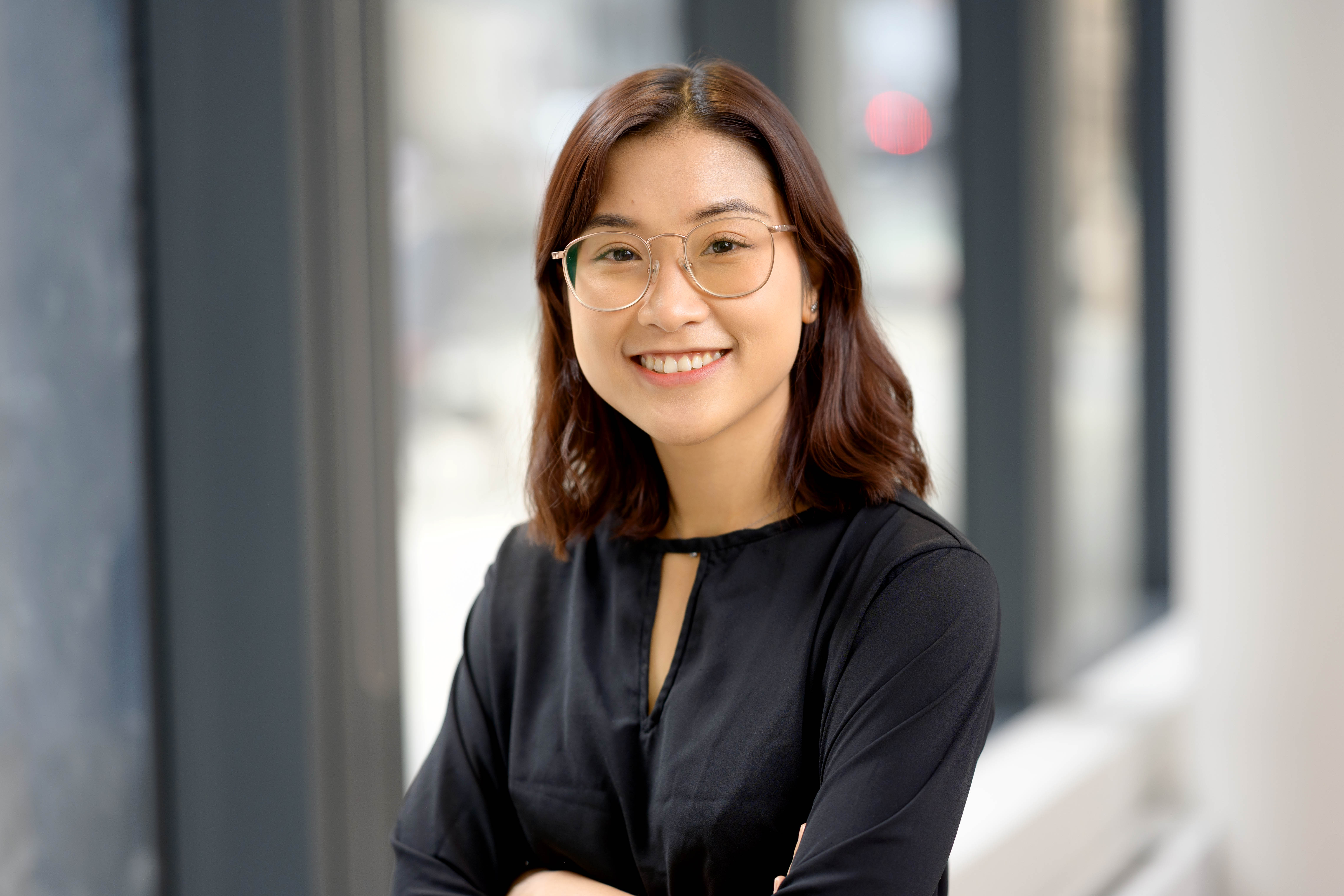
Thinh Tran
In my dissertation research in the lab of Nikolaus Schultz, PhD, I focused on leveraging real-world cancer data, encompassing clinical records and genomic data like MSK-IMPACT® panels. Despite its value, this data is often complex and difficult to utilize effectively.
One challenge I addressed was extracting treatment details from clinical notes, particularly when patients came to MSK from different institutions. To streamline this, I developed a natural language processing model that accurately identified whether patients received prior treatment elsewhere, making this information easily accessible for researchers. This work was done in collaboration with the Cancer Data Science Initiative’s Clinical Data Mining team and contributed to the creation of a richly annotated Clinicogenomic Harmonized Oncologic Real-world Dataset (MSK-CHORD). This dataset can adequately power discovery of clinicogenomic associations, including those among patients with less common characteristics.
Another focus was interpreting genetic variants in tumors, particularly those mutations with unknown significance that made up approximately 80% of detected mutations. Evaluating 11 computational methods known as variant effect predictors (VEPs), which leverage information about the evolutionary and structural contexts of the mutations to predict whether they are harmful, we that found AlphaMissense, a deep-learning method built on the protein-folding predictor AlphaFold2, was the most accurate at identifying known harmful mutations in cancer.
Validating AlphaMissense’s predictions in two cohorts of patients with lung cancer, we identified mutations in KEAP1 and SMARCA4 that are associated with poorer survival, suggesting their significance in cancer progression. This research validates computational tools for real-world outcomes, aiding in prioritizing mutations for further study.
Throughout my time at GSK, I was fortunate to receive invaluable mentorship and to collaborate with diverse interdisciplinary teams. Following my PhD, I will be joining the data insights team at Natera as a bioinformatic data scientist, where I will continue to work with large cancer datasets to uncover biological insights and potential biomarkers for improved cancer treatment.