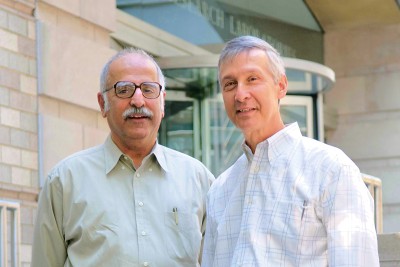
Dinshaw Patel (left) and David Allis
DNA’s Packaging Proteins Hold Clues to How Our Genes Work and What Causes Them to Malfunction
Structural biologists at Memorial Sloan Kettering Cancer Center are collaborating with biochemists and cell biologists at The Rockefeller University to study how cells read genetic instructions imprinted on histones, DNA’s packaging proteins. Their research has revealed new mechanisms by which some diseases, including certain types of leukemia, may arise.
“Without histones, our DNA would never fit inside a cell,” noted Dinshaw J. Patel, a Member in Sloan Kettering Institute’s Structural Biology Program and incumbent of the Abby Rockefeller Mauzé Chair of Experimental Therapeutics. Stretched out, the DNA of one human cell would be about six feet long. It fits inside the cell’s nucleus — which is only six micrometers in diameter — by wrapping around a series of spool-like structures, which are made of proteins called histones.
Wound up on these spools, each long DNA strand resembles a thick wire that coils and bends like a tangled-up telephone cord to shape a chromosome.
In the laboratory of Dr. Patel, who was elected earlier this year to the National Academy of Sciences, researchers are experts in visualizing the three-dimensional structure of a cell’s basic components, such as proteins and DNA. These components carry out all of our cells’ functions, and the way they fold into particular conformations, or shapes, is critical to how they work. For the past four years, working with C. David Allis — a Rockefeller University professor and Member Affiliate in SKI’s Molecular Biology Program — and colleagues, Dr. Patel’s structural biology team has been exploring DNA’s packaging proteins.
Indexing Genes
For almost half a century, histones were believed to do little else than pack DNA — compacting and organizing its long threads. But in the past two decades, scientists have learned that histones play many other roles in the life of a cell, such as carrying instructions about which genes should be active or silent (switched on or off) in any given cell at any given moment. “Research in this area by Dr. Allis and his co-workers has had a profound impact on the modern view of histones and their function,” Dr. Patel observed.
Because DNA is packed so tightly, most genes are inaccessible to the proteins that activate them and are kept in a silent state. Before a gene can be activated, the piece of DNA containing its genetic message must be loosened from its spools.
Almost a decade ago, Dr. Allis and colleagues proposed that the histone proteins within the spools carry a code that regulates the activity of our genes. Segments, or tails, of histone proteins protrude from the spools’ surfaces, and a variety of small chemical tags are constantly added to or removed from these protruding tails. How the histone tails are tagged determines if the DNA surrounding the spools will unwind, making genes more accessible for activation.
Throughout our chromosomes, histone tags create a dynamic indexing system for genes, like Post-it notes flagging pages in a catalog. This indexing system helps a cell establish its identity. There are about 200 types of cells in a human body, all of which carry the same DNA — the same catalog of genes. But each cell type uses only certain genes at certain times, allowing it to carry out its individual task (for instance, red blood cells carry oxygen, and nerve cells transmit electrical signals).
The way a cell regulates its genes determines its fate. For example, numerous genes need to be activated and silenced during normal growth and development so that cells divide, acquire new traits, or expire in a programmed manner. If cells fail to regulate these genes correctly, this can lead to developmental defects or cancer.
Exploring How the Tags Work
A variety of histone tags are scattered on DNA’s spools, with each tag carrying its own meaning. Some tags indicate when genes should be turned on, others when they should be turned off. There are even tags that can indicate both, depending on where they sit on a spool in relation to other tags. So how are all these tags added to and removed from the histones’ tails? And how can a cell “read” them and interpret their meaning?
“Thousands of proteins in our cells carry out these functions,” Dr. Patel explained. “There are proteins called writers that add tags, and erasers that remove them. And numerous reader proteins can recognize specific tags, or even combinations of tags.” The reader proteins are part of a more complex machinery of proteins, which facilitate either unwinding of the DNA so genes can be activated or keeping the DNA wrapped up.
In 2005, Dr. Allis and then-postdoctoral fellow Joanna Wysocka discovered how one type of tag — which acts as an on switch by flagging genes for activation — can be read and interpreted. To read this particular tag, known as histone H3 lysine 4 trimethylation, proteins often use a PHD finger within their structure. The PHD finger is what biologists call a protein module — a special segment of the protein that gives it the correct shape to carry out a specific task, such as binding to another molecule.
The Rockefeller researchers turned to Dr. Patel’s lab to decipher the molecular principles underlying the PHD finger’s ability to read a specific histone tag. “I believe every amino acid building block of a histone protein must be there for a reason,” Dr. Allis said. “With their elegant structural studies, the Patel team is verifying just how true this is. They work magic in their laboratory.”
Working such “magic,” Haitao Li, a senior research scientist in Dr. Patel’s laboratory, used a method called x-ray crystallography to visualize a protein’s PHD finger. Based on his findings, the investigators could determine precisely how the protein binds to the gene-activating tag on a histone spool, and how it distinguishes it from other types of tags.
On Dr. Patel’s computer screen, a three-dimensional model of the PHD finger reading the tag appears as a mass of knobs, balls, and sticks. The tag and molecules surrounding it are snugly accommodated in adjacent pockets of the PHD finger, like a key fitting its lock.
To create this model, Dr. Li first had to isolate the protein in its purest possible form and then coax it into forming a crystal, in which all the protein’s atoms are arranged in an orderly lattice.
Next, he bombarded the crystal with high-energy x-rays, which diffract or bend in defined directions as they interact with atoms within the crystal. As the rays exit the crystal, they form a unique pattern that can be captured as an image. The pattern indicates how individual atoms are arranged inside the crystal.
Dr. Li captured a stack of x-ray images, each taken from a different angle. He then compiled them using software to calculate a three-dimensional image of the protein, similarly to the way in which a CT scan makes an image of a person’s body or tissue.
Misinterpretation and Cancer

X-ray crystallography model of a PHD finger reading a histone tag
Determining a protein’s structure is often a critical step toward understanding its function — in normal cells and tissues or in disease. “Sometimes when you solve a protein’s structure, the answer to its biological function just leaps out,” noted Dr. Patel.
By determining the shape of the PHD finger and how it binds to a specific histone tag, the Memorial Sloan Kettering-Rockefeller team clarified how some of the messages on DNA’s spools are read so that genes can be activated. More than 200 proteins in our cells have this module in their structure and are likely to be involved in numerous biological processes.
Recently, the team discovered that reader proteins with a PHD finger could contribute to some types of cancer. Published in Nature this June, their study has revealed a new mechanism underlying acute myeloid leukemia, in which cells misinterpret the histone’s messages.
In many leukemia patients, two separate genes fuse together. Occasionally, this gene alteration can lead to the production of a malfunctioning protein called NUP98-JARID1A. The aberrant protein has a PHD finger that is placed out of context.
Gang Wang, a postdoctoral fellow in Dr. Allis’ laboratory, examined the aberrant protein in leukemia mouse models. He discovered that its production has severe consequences for the development of blood cells, and also makes the cells become cancerous. Because blood stem cells fail to regulate certain genes, they cannot mature into functional blood cells, such as immune cells. This also makes the cells unable to stop dividing, which is a hallmark of cancer.
The researchers suspected that the cells were not interpreting their histone’s messages correctly. Their suspicions were confirmed once the structure of NUP98-JARID1A’s PHD finger was visualized by postdoctoral fellows Zhanxin Wang and Jikui Song in Dr. Patel’s laboratory.
“It’s really the PHD finger that causes the cancer,” explained Gang Wang, who confirmed this conclusion using his mouse models. The module makes the aberrant protein turn a number of genes on, but also prevents these genes from being silenced again — as if their activation switches have been jammed in the on position. “In a sense, the PHD finger makes cells misinterpret the histone tags,” he emphasized.
The Memorial Sloan Kettering-Rockefeller team believes similar mechanisms could account for other diseases. Gene mutations that in one way or another alter PHD fingers have been linked to several types of cancer, as well as some immune and neurological disorders.
The Next Challenge for Cancer Research
There is accumulating evidence that the histones’ messages present a gateway to understanding and treating disease. In a range of human cancers, genes have been shown to be regulated abnormally due to alterations in the proteins that write, erase, or read histone tags. New therapies have also been designed to adjust the histones’ messages when they have gone awry. One family of drugs, called histone deacetylase (HDAC) inhibitors, has proven to be effective for treating several types of cancer.
“The implications of histones and their dynamic messages are only beginning to be appreciated in clinical oncology,” Dr. Patel noted. For example, he and his colleagues believe that each message may be composed of several tags sitting together on DNA’s spools, like letters forming words and sentences. Dr. Li of Memorial Sloan Kettering and postdoctoral fellow Alexander Ruthenburg of Rockefeller are examining how different tags on a spool are read in combination. “A major challenge for future research and therapy will be to understand how the histones’ messages are read in the context of a living cell, rather than in a test tube,” Dr. Patel added.
Dr. Li is also working with postdoctoral fellow Andrew Xiao of Rockefeller to explore how DNA’s spools are tagged in places where the DNA has been damaged — another possible link between histones and cancer. Their initial findings, also published in Nature, in January, have clarified how certain types of histones instruct cells to deal with DNA damage — by repairing their broken DNA or by undergoing cell death. This “choice” is critical for the fate of a cell, since failures in DNA repair can lead to genetic alterations and cancer.
“We suspect that histone spools have many more secrets to share, with profound implications for human biology and disease,” Dr. Patel said. He and Dr. Allis expect their collaboration will continue to lead them into new and serendipitous areas of research. “Time and time again,” Dr. Allis observed, “we find that our two-laboratory team is a successful ’marriage,’ where the sum is greater than the parts.”